Massive Gravity and the end of dark energy
By Claudia de Rham | Theoretical physicist working at the interface of gravity, cosmology, and particle physics at Imperial College London.
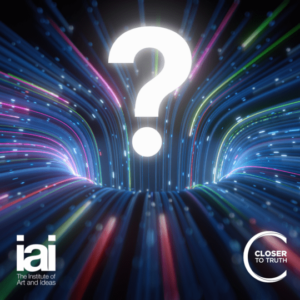
This article was created as part of a series in partnership with The Institute of Art and Ideas. To explore more articles from the series and a fantastic range of articles, videos and more from The Institute of Art and Ideas, head over to IAI.TV.
The beauty of cosmology is that it often connects the infinitely small with the infinitely big—but within this beauty lies the biggest embarrassment in the history of physics.
According to Einstein’s theory of General Relativity and our knowledge of particle physics, the accumulated effect of all infinitely small quantum fluctuations in the cosmos should be so dramatic that the Universe itself should be smaller than the distance between the Earth and the Moon. But as we all know, our Universe spans over tens of billions of light years: it clearly stretches well beyond the moon.
This is the “Cosmological Constant Problem”. Far from being only a small technical annoyance, this problem is the biggest discrepancy in the whole history of physics. The theory of Massive Gravity, developed by my colleagues and I, seeks to address this problem.
For this, one must do two things. First, we need to explain what leads to this cosmic acceleration; second, we need to explain why it leads to the observed rate of acceleration—no more no less. Nowadays it is quite popular to address the first point by postulating a new kind of Dark Energy fluid to drive the cosmic acceleration of the Universe. As for the second point, a popular explanation is the anthropic principle: if the Universe was accelerating at a different rate, we wouldn’t be here to ask ourselves the questions.
To my mind, both these solutions are unsatisfactory. In Massive Gravity, we don’t address the first point by postulating a new form of as yet undiscovered Dark Energy but rather by relying on what we do know to exist: the quantum nature of all the fundamental particles we are made out of, and the consequent vacuum energy, which eliminates the need for dark energy. This is a natural resolution for the first point and would have been adopted by scientists a long time ago if it wasn’t for the second point: how can we ensure that the immense levels of vacuum energy that fill the Universe don’t lead to too fast an acceleration? We can address this by effectively changing the laws of gravity on cosmological scales and by constraining the effect of vacuum energy.
The Higgs Boson and the Nature of Nothingness
At first sight, our Universe seems to be filled with a multitude of stars within galaxies. These galaxies are gathered in clusters surrounded by puffy “clouds” of dark matter. But is that it? Is there anything in between these clusters of galaxies plugged in filaments of dark matter? Peeking directly through our instruments, most of our Universe appears to be completely empty, with empty cosmic voids stretching between clusters of galaxies. There are no galaxies, nor gas, nor dark matter nor anything else really tangible we can detect within these cosmic voids. But are they completely empty and denuded of energy? To get a better picture of what makes up “empty space,” it is useful to connect with the fundamental particles that we are made of.
I still vividly remember watching the announcement of the discovery of the Higgs boson in 2012. By now most people have heard of this renowned particle and how it plays an important role in our knowledge of particle physics, as well as how it is responsible for giving other particles mass. However, what’s even more remarkable is that the discovery of the Higgs boson and its mechanism reveals fundamental insights into our understanding of nothingness.
To put it another way, consider “empty space” as an area of space where everything has been wiped away down to the last particle. The discovery of the Higgs boson indicates that even such an ideal vacuum is never entirely empty: it is constantly bursting with quantum fluctuations of all known particles, notably that of the Higgs.
This collection of quantum fluctuations I’ll refer to as the “Higgs bath.” The Higgs bath works as a medium, influencing other particles swimming in it. Light or massless particles, such as photons, don’t care very much about the bath and remain unaffected. Other particles, such as the W and Z bosons that mediate the Weak Force, interact intensely with the Higgs bath and inherit a significant mass. As a result of their mass the Weak Force they mediate is fittingly weakened.
Accelerating Expansion
When zooming out to the limits of our observable Universe we have evidence that the Universe is expanding at an accelerating speed, a discovery that led to the 2011 Nobel Prize in Physics. This is contrary to what we would have expected if most of the energy in the Universe was localized around the habitable regions of the Universe we are used to, like clusters of galaxies within the filaments of Dark Matter. In this scenario, we would expect the gravitational attraction pulling between these masses to lead to a decelerating expansion.
So what might explain our observations of acceleration? We seem to need something which fights back against gravity but isn’t strong enough to tear galaxies apart, which exists everywhere evenly and isn’t diluted by the expansion of the cosmos. This “something” has been called Dark Energy.
A different option is vacuum energy. We’ve long known that the sea of quantum fluctuations has dramatic effects on other particles, as with the Higgs Bath, so it’s natural to ask about its effect on our Universe. In fact, scientists have been examining the effect of this vacuum energy for more than a century, and long ago realized that its effects on cosmological scales should lead to an accelerated expansion of the Universe, even before we had observations that indicated that acceleration was actually happening. Now that we know the Universe is in fact accelerating, it is natural to go back to this vacuum energy and estimate the expected rate of cosmic acceleration it leads to.
The bad news is that the rate of this acceleration would be way too fast. The estimated acceleration rate would be wrong by at least twenty-eight orders of magnitude! This is the “Cosmological Constant Problem” and is also referred to as the “Vacuum Catastrophe.”
Is our understanding of the fundamental particles incorrect? Or are we using Einstein’s theory of General Relativity in a situation where it does not apply?
The Theory of Massive Gravity
Very few possibilities have been suggested. The one I would like to consider is that General Relativity may not be the correct description of gravity at large cosmological scales where gravity remains untested.
In Einstein’s theory of gravity, the graviton like the photon is massless and gravity has an infinite reach. This means objects separated by cosmological scales, all the way up to the size of the Universe, are still under the gravitational influence of each other and of the vacuum energy that fills the cosmos between them. Even though locally the effect of this vacuum energy is small, when you consider its effect accumulated over the whole history and volume of the Universe, its impact is gargantuan, bigger than everything else we can imagine, so that the cosmos would be dominated by its overall effect. Since there is a lot of vacuum energy to take into account, this leads to a very large acceleration, much larger than what we see, which again is the Cosmological Constant Problem. The solution my colleagues and I have suggested is that perhaps we don’t need to account for all this vacuum energy. If we only account for a small fraction of it, then it would still lead to a cosmic acceleration but with a much smaller rate, compatible with the Universe in which we live.
Could it be that the gravitational connection that we share with the Earth, with the rest of the Galaxy and our local cluster only occurs because we are sufficiently close to one another?
Could it be that we do not share that same gravitational connection with very distant objects, for instance with distant stars located on the other side of the Universe some 10 thousand million trillion km away? If that were the case, there would be far less vacuum energy to consider and this would lead to a smaller cosmic acceleration, resolving the problem.
In practice, what we need to do is understand how to weaken the range of gravity. But that’s easy: nature has already showed us how to do that. We know that the Weak Force is weak and has a finite range distance because the W and Z bosons that carry it are massive particles. So, in principle, all we have to do is simply to give a mass to the graviton. Just as Einstein himself tried to include a Cosmological Constant in his equations, what we need to do is add another term which acts as the mass of the graviton, dampening the dynamics of gravitational waves and limiting the range of gravity. By making the graviton massive we now have ourselves a theory of “massive gravity.” Easy!
The possibility that gravity could have a finite range is not a question for science-fiction.
In fact Newton, Laplace and many other incredible scientists after them contemplated the possibility. Even following our development of quantum mechanics and the Standard Model, many including Pauli and Salam considered the possibility of gravitons with mass.
But that possibility was always entirely refuted! Not because it potentially contradicts observations – quite the opposite, it could solve the vacuum catastrophe and explain why our Universe’s expansion is accelerating – but rather because models of massive gravity appeared to be haunted by “ghosts.” Ghosts are particles with negative energies that would cause everything we know, including you, me, the whole Universe, and possibly the structure of space and time to decay instantaneously. So if you want a theory of massive gravity you need to either find a way to get rid of these ghosts or to “trap” them.
For decades, preventing these supernatural occurrences seemed inconceivable. That’s until Gregory Gabadadze, Andrew Tolley and I found a way to engineer a special kind of “ghost trap” that allowed us to trick the ghost to live in a constrained space and do no harm. One can think of this like an infinite loop-Escherian impossible staircase in which the Ghosts may exist and move but ultimately end up nowhere.
Coming up with a new trick was one thing, but convincing the scientific community required even more ingenuity and mental flexibility. Even in science, there are many cultures and mathematical languages or scientific arguments that individuals prefer. So, throughout the years, whenever a new colleague had a different point of view, we were bound to learn their language, translate, and adjust our reasoning to their way of thinking. We had to repeat the process for years until no stone remained unturned. Overcoming that process was never the goal, though: it was only the start of the journey that would allow us to test our new theory of gravity.
If gravitons have a mass, this mass should be tiny, smaller than the mass of all the other massive particles, even lighter than the neutrino.
Consequently, detecting it may not be straightforward. Nevertheless, different features will appear which may make it possible to measure it.
The most promising way to test for massive gravity involves observations of gravitational waves. If gravitons are massive, then we’d expect that low-frequency gravitational waves will travel ever so slightly slower than high-frequency ones. Unfortunately, this difference would be too slight to measure with current ground-based observatories. However, we should have better luck with future observatories. Missions like the Pulsar Timing Array, LISA and the Simons Observatory will detect gravitational waves with smaller and smaller frequencies, making possible the observations we need.
Whether the massive gravity theory developed by my collaborators and I will survive future tests is of course presently unknown, but the possibility is now open. After all, even if the outcome isn’t certain, when it comes to challenging the biggest discrepancy of the whole history of science, addressing the Cosmological Constant Problem, eliminating the need for dark energy, and reconciling the effect of vacuum energy with the evolution of the Universe, some risks may be worth taking.
Claudia de Rham has recently published her first book, The Beauty of Falling: A Life in Pursuit of Gravity, with Princeton University Press. De Rham reveals how great minds—from Newton and Einstein to Stephen Hawking, Andrea Ghez, and Roger Penrose—led her to the edge of knowledge about this fundamental force.